Microfractures or bone marrow stimulation (BMS): evolution of the technique
Microfracturas o estimulación de la médula ósea (BMS): la evolución de la técnica
Resumen:
La microfractura es la técnica más utilizada en la reparación de las lesiones condrales focales de las articulaciones. En los últimos años, la técnica ha mejorado con la incorporación de la artroscopia, la mejora del instrumental utilizado y, últimamente, por participar de los adelantos de la ingeniería de tejidos. En las llamadas microfracturas plus, los nuevos biomateriales rellenan la lesión y retienen el sangrado permitiendo que se forme un coágulo, con todo el material biológico incorporado, que permite un proceso de reparación más biológico, con un mejor resultado estructural y, por lo tanto, con un tejido que soporta mejor las solicitaciones mecánicas y permite una mayor duración. Hacemos una revisión de la situación actual de algunas de estas técnicas.
Abstract:
Microfracture is the most widely used technique for repairing focal chondral lesions of the joints. In recent years, the procedure has been improved with the incorporation of arthroscopy, optimization of the instruments used and, lately, the introduction of advances in the field of tissue engineering. In the so-called microfracture plus method, novel biomaterials are used to fill the lesion and retain bleeding to thus form a clot with all the incorporated biomaterial component - resulting in a more biological repair process with improved structural outcomes, and affording a tissue better able to support the mechanical demands with increased durability. A review is made of the state of the art of some of these techniques.
Introduction
Microfractures are actually an evolution of the original technique developed by Pridie in the 1950s(1) and improved upon by Steadman in the 1980s(2). This technique has become increasingly popular over the years, to the point where it now constitutes the reference for cartilage repair and the technique against which other procedures are compared. With all its variants, it remains the most widely used technique for repairing focal chondral lesions(3).
Since joint cartilage is a tissue without innervation or vascularization in most of its structure, it lacks the intrinsic capacity to generate an adequate response to tissue damage causing alteration of its structure, with local bleeding. The creation of subchondral bone perforations causes active bleeding from the bone marrow and from the microvascularization that perforates the subchondral plate, with penetration into the calcified layer of the cartilage. Such bleeding gives rise to a clot ("superclot" according to Steadman) that is formed in an environment which from the mechanical perspective is very hostile to it, and which can cause it to easily become detached before being able to complete its function(4).
However, the microfractures technique in fact comprises a series of techniques grouped under the term of bone marrow stimulation (BMS). The techniques based on BMS have evolved over the years. Thus, the methodology has progressed from simple abrasion to large-calibre motorized perforations and non-motorized "ice pick" perforations (Figure 1), measuring between 1.5-2.5 mm in diameter, and finally to techniques that use small-calibre perforations with depths of up to 9 mm that are much less aggressive with the bone and marrow structure, and are referred to as subchondral drilling techniques.
Along with this technical evolution, a number of strategies have been added to cover and protect the blood clot as the basic component for starting the healing process, with a view to improving the outcomes. This is what is known as “microfracture plus”, enhanced BMS or also augmented microfracture(5,6).
The present article describes the biological principles on which these procedures are based, as well as some of the technologies that are used and which base their mechanism of action on principles we consider to be physiologically "elegant".
Biological principles
This series of techniques is based on biological principles, since the presence of the blood clot is essential for triggering the complex healing process in all human tissues. The mentioned blood clot contains all the elements needed to produce, in successive stages, the cascade of events - induction of the reparatory inflammatory response, followed by modulation of the latter and appearance of the new tissue through maturation of the granulation tissue - in a natural and coordinated manner, to finally give rise to the healing tissue. The latter may be identical to the original tissue (restitutio ad integrum), and in this case can technically be referred to as "regenerated tissue", or it may constitute a scar of variable quality depending on the degree to which it resembles the original tissue - in which case we speak of "repaired tissue".
In the immense majority of cases, joint cartilage repair results in healing tissue of variable quality depending on its hydration and the presence, orientation and percentage of type II collagen, which is characteristic of joint cartilage and is also referred to as hyaline cartilage. If this type of collagen is absent or is present in less than normal proportions, we speak of fibrocartilage. However, we prefer to use the term cartilage with more or less "hyaline characteristics", since this is closer to what is seen in the real life scenario. The more hyaline the cartilage obtained, i.e., the more stratified the tissue, with the presence of type II collagen in adequate amounts and locations, with correct hydration as evidenced by the presence of glycosaminoglycans (GAGs) and with adequate thickness, the better and more lasting the outcomes are expected to be. In contrast, when the presence of type I collagen increases versus the presence of type II collagen, with non-stratified or excessively or deficiently hydrated tissue, the outcome is a fibrous tissue (fibrocartilage) with inferior biomechanical performance, unable to perform its dual physiological function as a sliding surface and distributor of mechanical forces, and accelerated deterioration will thus result.
Since joint cartilage is a tissue without innervation or vascularization in most of its structure (but not in all of its structure, as we will see below), it lacks the intrinsic capacity to generate an adequate response to tissue damage causing a substantial alteration of its structural integrity, with local bleeding. The creation of subchondral bone perforations communicating the joint environment with the subchondral space causes active bleeding from the bone marrow and from the microvascularization that perforates the subchondral plate, with penetration into the calcified layer of the cartilage. Such bleeding gives rise to a clot ("superclot" according to Steadman) that is formed in an environment which from the mechanical perspective is very hostile to it, and which can cause it to easily become detached before being able to adequately complete its function.
The blood clot as primary factor
The blood clot has a number of functions, all of which are crucial, in the tissue healing process. However, damage to a vascularized tissue results in rupture of the local blood vessels. This in turn triggers a chain of perfectly coordinated events. Coagulation is the first event, and seeks to arrest bleeding as quickly as possible, since hemorrhage ultimately can threaten survival of the tissue.
The clot constitutes a biologically active three-dimensional barrier composed of fibrin and platelets. The platelets have two functions: a purely hemostatic function in which they accumulate at the walls of the ruptured vessel in an attempt to seal the perforation; and a secretory function upon becoming activated, characterized by immediate release of the elements contained in their "alpha" granules. In this way a number of cytokines are secreted, such as PDGF (platelet derived growth factor) and TGF-β (transforming growth factor beta), which contribute to induce an inflammatory response. In a second step, other similar substances such as VEGF (vascular endothelial growth factor) and FGF (fibroblast growth factor), among many others, modulate the response and the start of the repair activity (healing)(7).
Depending on the tissue involved, healing may be more or less sophisticated, ranging from simple and fibrous healing to structured repair with restitutio ad integrum of the damaged tissue - as occurs in the case of bone tissue.
Influences from subchondral bone
As its name indicates, subchondral bone is the zone underlying the joint cartilage, more specifically the calcified layer of the cartilage, with which it forms a "functional unit" with the rest of the cartilage(8). Subchondral bone constitutes the biomechanical transition between cartilage properly speaking - the role of which is to secure minimum friction and distribute the mechanical forces to which it is exposed - and the bone. The mechanical forces are absorbed by the subchondral bone and in turn are transmitted to the metaphysis and diaphysis of the bone.
The contact surface between cartilage and bone is not flat but rather wavy - thus affording a greater contact area and a very effective inter-blocking effect.
On the other hand, the subchondral region is highly vascularized, with arteriovenous microvascularization and a certain nerve supply. These structures penetrate through microcanals to reach the calcified layer of the cartilage. This has implications in the event of injury(9) and partly explains why full-thickness chondral lesions (corresponding to grade 4 of the International Cartilage Repair Society [ICRS] and Outerbridge score) are able to generate a spontaneous repair response, and also why BMS procedures (such as the microfractures technique) yield good (albeit not optimum) results. In addition, these features explain the need for curettage of the calcified layer of cartilage with these techniques, since its removal causes bleeding(10).
Influences from the synovial membrane
The chondrogenic process produced by the synovial membrane has received less attention than that associated with the subchondral bone. However, the mesenchymal cells from the synovial membrane have been shown to have a "tremendous chondrogenic potential"(11). In comparison with other sources such as the bone marrow or adipose tissue, these cells have shown to have a greater capacity to contribute to the chondrogenic process(12). This is not surprising, since the synovial membrane is well known for its role in maintaining joint homeostasis. The synovial membrane is richly innervated and vascularized - these being two important factors for producing a reparatory inflammatory response. For this reason, any implant placed over a focal cartilage lesion must be sufficiently porous to allow the penetration of chondrogenic cells and bioactive substances(13).
Microfracture plus
The results obtained with the microfracture techniques show variations, depending on the consulted literature source. This is due to the different hyaline characteristics of the regenerated cartilage. In some cases the percentage of fibrocartilage is high (Figure 2), due to a greater presence of type I collagen and the absence of type II collagen, in addition to the absence of a stratified cartilage tissue. Hyaline cartilage is characterized by the presence of type I collagen at the sliding surface, while type II collagen is the predominant form in the rest of the tissue, distributed to conform three basic stratification zones. These structural characteristics confer the biomechanical properties that allow long lasting function(13).
Experimental studies involving different microfracture augmentation techniques, as well as clinical studies, consistently report different degrees of improvement in the amount and quality (hyaline characteristics) of the new tissue, which should result in longer duration of the latter as well as afford improved clinical outcomes(14).
The basic biological principles are the same as those mentioned in reference to microfractures. Protection of the structure responsible for the blood clot is added, however, employing different natural biomaterials (collagens, alginates, fibrins, hyaluronic acid, hydroxyapatite, chitosan) and synthetic materials (polyglycolic and polylactic acids in their different variants), implanted in different formats (solid matrixes, membranes, gels). This contributes to the repair process.
As pointed out by Brittberg(15), one of the pioneers in chondrocyte culture technology, cells are always needed to repair tissue damage through biological means. What changes is the way in which they are used. One option is to attract the cells towards the lesion site by means of "something" that amplifies the physiological response - what Brittberg refers to as "intrinsic" use of the cells (in this case "in situ chondroinduction"), while another option is to grow the cells in culture and subsequently implant them ("extrinsic" use). Both options are valid, of course, though there may be indications where one is more appropriate than the other, based on aspects such as patient age, activity and height, as well as lesion depth or location. It is also necessary to consider aspects such as the cost-benefit ratio and surgeon experience when deciding to use one option or another.
This cellular substrate, which is not the topic of the present review, is referred to in several ways. The most common term is mesenchymal stem cells (MSCs)(16), renamed by Caplan(17) who initially described them in the late 1980s as medicinal signaling cells, since this term reflects their nature and action better. The group led by Caplan called for reflection upon the challenges posed by the chondrogenic differentiation of this cell line, particularly when believing that these cells alone are able to form hyaline cartilage(18). As orthopedic surgeons, our actions must favour and facilitate the functioning of this system, though in no case should we attempt to modify it, since this is where we can run into greater problems.
With the microfracture plus technique, the aim is to generate this cell population and the rest of the bioactive components that intervene in chondrogenesis, creating an optimum environment within the "synovial organ" (which is how synovial joints should be understood - with all which this term implies) in order for the process to develop as best as possible, and countering the negative influences derived from the hostile microenvironment which a joint represents.
Materials used
We do not intend to conduct an exhaustive review of the different material options and of some of the products currently available for improving the performance of microfractures. The key is to understand the rationale of the underlying mechanism of action. The choice of one technology or another depends on the surgeon. All the technologies based on these principles have something to offer when conceived on the basis of the described biological principles, with correct application of the latter. The classification that follows below is arbitrary.
AMIC membranes
The term "AMIC" refers to "autologous matrix-induced chondrogenesis". It was initially used in reference only to the utilization of a specific two-layer matrix of porcine origin composed of type I and type III collagen (Chondro-Gide®, Geistlich Biomaterials AG, Wolhusen-Switzerland)(19,20). In fact, AMIC® is a registered trademark of Geistlich, and the term theoretically should only be used in procedures that use this particular matrix. However, its use has also spread to other options, and we now speak of "AMIC-type" procedures. It has even been used concomitant to the implantation of autologous chondrocytes to seal the lesion and contain the chondrocytes in suspension(21).
As with all microfracture plus procedures, the chosen implant (in this case the membrane) seeks to protect the structural and functional integrity of the clot produced from BMS and, if possible, favour its action. In order to stabilize the membrane, the latter must be applied with fibrin glue at the margins in contact with the surrounding cartilage.
The most recent review related to this technique(22) analyses the results of 12 studies on focal chondral lesions of the knee. One of these studies involved a randomized, comparative design, with 375 patients. The study describes positive outcomes over the middle term (up to 5 years) in terms of both the clinical appearance and the structure of the new tissue. Sixty-six percent of the patients presented good repair quality as determined using semi-quantitative methods such as MOCART (Magnetic Resonance Observation of Cartilage Repair Tissue). This technique has also been used in joints other than the knee, with positive results.
Other membranes can be found on the market, such as the widely used Hyalofast® (Anika Therapeutics, Padova, Italy), made from a hyaluronic acid ester base. More solid three-dimensional implants are also available, such as for example MaioRegen® (Finceramica, Faenza, Italy), made from type I collagen and hydroxyapatite in a stratified structure that is also useful for bone regeneration, as in patients with lesions characterized by an important osteochondral component. Another option is Agili-C™ (CartiHeal Ltd., Ariel, Israel), manufactured from aragonite (calcium carbonate derived from sea coral). At present, a randomized study is ongoing in the United States, comparing this product versus the standard of care, for presentation to the Food and Drug Administration (FDA) with the aim of obtaining its approval.
Gels and similar products
This section includes GelrinC® (Regentis Biomaterials, Akiva, Israel), based on a mixture of polyethylene glycol diacrylate (PEG-DA) and denaturalized fibrinogen, that reacts upon being exposed to ultraviolet light, with transformation into a semisolid gel. A comparative study with microfracture alone is also ongoing at this time in the United States, with a view to obtaining approval of the product. Another option among the hydrogels is CartiFill® (Sewon Cellontech, South Korea), based on atherocollagen, and which has been in use for some years in several countries (Figure 3).
One of the most widely known and studied materials, and to date the only material included in randomized clinical trials, is chitosan.
Chitosan
Chitosan is a natural polysaccharide derived from chitin; it is the second most abundant biological material in nature after cellulose. Chitosan is well known for its medical uses, in stimulating healing, and as antimicrobial and antihemorrhagic treatment, among other applications. It therefore was viewed as an attractive material for use in tissue engineering. It application in orthopedics is relatively recent, however.
The chemical structure of chitosan is very similar to that of the glycosaminoglycans (GAGs) (chondroitin sulfate and keratan sulfate), which are very important in hyaline cartilage structure and key elements for understanding its biomechanical behaviour. In addition, chitosan is able to interact with these substances and serve as a support for the production of aggrecan and type II collagen. This makes it a very attractive material for joint cartilage repair(23). On the other hand, chitosan adheres to the surface; no additional glue or adhesive is therefore needed.
Despite these undeniable and proven benefits, however, chitosan is very unstable, and the elaboration of products containing it therefore poses major challenges. A very interesting property of this biopolymer is the fact that when in liquid state, it transforms into a semisolid gel upon reaching human body temperature(24). This discovery was very useful in searching for a formulation that may be injected into a joint such as the knee in the course of an arthroscopic procedure, with solidification in situ in only a few minutes. The decision was therefore made to use it as a coadjuvant in BMS procedures. In 2005, the Canadian company BioSyntech – posteriorly Piramal Healthcare (Canada), Bio-Orthopedics Division – started an international, randomized, comparative clinical study contrasting microfractures alone with microfracture plus versus their product, BST-CarGel® (currently Smith & Nephew, Wartford, UK). The results after both 12 months and at 5 years of follow-up evidenced sustained superiority over time of BST-CarGel® versus microfractures alone. This superiority was reflected by both the greater hyaline quality and quantity of the formed tissue, as measured objectively using quantitative magnetic resonance imaging (MRI) techniques(25,26) (Figure 4).
reaca.28171.fs2002014en-figure4.png
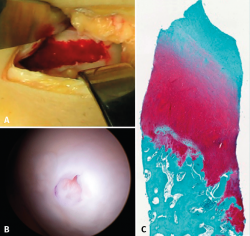
Figure 4. A: extensive chondral lesion of the internal condyle covered by the clot after augmented microfractures with chitosan based hydrogel; B: arthroscopic appearance of the totally covered zone and biopsy sampling orifice after 13 months; C: histological appearance of the biopsied zone. Note the "hyaline" appearance of the tissue and good interdigitation with the subchondral bone. Image of the randomized study with BST-CarGel.
A posterior version of this technology, developed by the same team, appeared in 2013, in the form of JointRep® (Oligomedic Inc., Laval, Canada). This improved version makes use of a formulation with a firmer consistency, reaching gel state in under two minutes - thus facilitating arthroscopic implantation and shortening the surgery time. A comparative study on microfractures in 69 patients and involving a follow-up period of two years recorded substantial clinical improvement as measured by the WOMAC (Western Ontario and McMaster Universities Osteoarthritis Index) versus the control group(27). An international, randomized, comparative multicentre study in Australia, New Zealand and Canada was in the preparatory phase in late 2020.
Conclusions
A procedure as simple and widely used in medical practice over many years as the creation of a lesion zone to induce bleeding - as in BMS based procedures - can be complemented by tissue engineering tools with a view to favouring or even amplifying the biological response necessary for tissue repair in hyaline joint cartilage, and thus secure even better outcomes and in a more consistent manner. This is what is achieved with microfracture plus. Given the available options for improving the success rates with bone marrow stimulation "augmentation" processes, the regular use of some of such strategies is fully justified in order to improve the outcomes and maintain them for longer periods of time. This leads us to ask ourselves one final question: Based on the existing evidence, is the use of microfractures alone a desirable strategy?
Figuras
Figure 2. A: arthroscopic appearance of an ulcer with bleeding microfracture orifices; B: appearance of the same zone covered by fibrocartilage 8 months later.
Figure 4. A: extensive chondral lesion of the internal condyle covered by the clot after augmented microfractures with chitosan based hydrogel; B: arthroscopic appearance of the totally covered zone and biopsy sampling orifice after 13 months; C: histological appearance of the biopsied zone. Note the "hyaline" appearance of the tissue and good interdigitation with the subchondral bone. Image of the randomized study with BST-CarGel.
Información del artículo
Cita bibliográfica
Autores
Alberto Restrepo Betancourt
Chief Medical Officer. OligoMedic Inc. Canadá
Ethical responsibilities
Conflicts of interest. The author Alberto Restrepo is chief medical officer of OligoMedic Inc.
Financial support. This study has received no financial support.
Protection of people and animals. The authors declare that this research has not involved studies in humans or in animals.
Data confidentiality. The authors declare that the protocols of their centre referred to the publication of patient information have been followed.
Right to privacy and informed consent. The authors declare that no patient data appear in this article.
Referencias bibliográficas
-
1Insall JN. Intra-articular surgery for degenerative arthritis of the knee. A report of the work of the late K. H. Pridie. J Bone Joint Surg (Br). 1967;49-B:211-28.
-
2Steadman JR, Rodkey WG, Rodrigo JJ. Microfracture: surgical technique and rehabilitation to treat chondral defects. Clin Orthop Relat Res. 2001;391(Suppl):S362-9.
-
3McCormick F, Harris JD, Abrams GD, Frank R, Gupta A, Hussey K, et al. Trends in the Surgical Treatment of Articular Cartilage Lesions in the United States: an Analysis of a Large Private-Payer Database Over a Period of 8 Years. Arthroscopy. 2014;30:222-6.
-
4Steadman JR, Rodkey WG, Briggs KK. Microfracture: Its History and Experience of the Developing Surgeon. Cartilage. 2010;1:78-86.
-
5Arshi A, Fabricant PD, Go DE, Williams RJ, McAllister DR, Jones KJ. Can Biologic Augmentation Improve clinical outcomes following microfracture for symptomatic cartilage defects of the knee? A systematic review. Cartilage. 2018;9:146-55.
-
6Gomoll AH. Microfracture and augments. J Knee Surg. 2012;25:9-16.
-
7Vaquero J, Forriol F. Knee chondral injuries: clinical treatment strategies and experimental models. Injury 2012;43:694-705.
-
8Imhof H, Sulzbacher I, Grampp S, Czerny C, Youssefzadeh S, Kainberger F. Subchondral Bone and Cartilage Disease: a Rediscovered Functional Unit. Invest Radiol. 2000;35:581-8.
-
9Madry H, van Dijk CN, Mueller-Gerbl M. The Basic Science of the Subchondral Bone. Knee Surg Sports Traumatol Arthrosc. 2010;18:419-33.
-
10Frisbie DD, Morissete S, Ho CP, Rodkey WG, Steadman JR, McIlwraith CW. Effects of calcified cartilage on healing of chondral defects treated with microfracture in horses. Am J Sports Med. 2006;34:1824-31.
-
11Hunziker EB. Articular cartilage repair: basic science and clinical progress. A review. Osteoarthritis Cartilage. 2001;10:432-63.
-
12Sakaguchi Y, Sekiya I, Yagishita K, Muneta T. Comparison of Human Stem Cells derived from various mesenchymal tissues: superiority of synovium as a cell source. Arthritis Rheum. 2005;52:2521-9.
-
13Bravo B, Guisasola MC, Vaquero J, Tirado I, Gortazar AR, Forriol F. Gene expression, protein profiling, and chemotactic activity of infrapatellar fat pad mesenchymal stem cells in pathologies of the knee joint. J Cell Physiol. 2019;234:18917-27.
-
14Strauss EJ, Barker JU, Kercher JS, Cole BJ, Mithoefer K. Augmentation strategies following the Microfracture technique for repair of focal chondral defects. Cartilage. 2010;1:145-52.
-
15Brittberg M. Cellular and Acellular approaches for cartilage repair: a philosophical analysis. Cartilage. 2015;6(Suppl 2):4S-12S.
-
16Caplan AI. Mesenchymal Stem Cells: The Past, the Present, the Future. Cartilage. 2010;1:6-9.
-
17Caplan AI. Mesenchymal Stem Cells: Time to Change the Name! Stem Cells Trans Med. 2017;6:1445-51.
-
18Somoza RA, Welter JF, Correa D, Caplan AI. Chondrogenic differentiation of Mesenchimal Stem Cells: challenges and unfulfilled expectations. Tissue Eng Part B. 2014;20:596-608.
-
19Benthien JP, Behrens P. Autologous Matrix-Induced Chondrogenesis (AMIC). Combining microfracturing and a collagen I/III matrix for articular cartilage resurfacing. Cartilage. 2010;1:65-8.
-
20Lee YH, Suzer F, Thermann H. Autologous Matrix-Induced Chondrogenesis: a review. Cartilage. 2014;5:145-53.
-
21Steinwachs MR, Kreuz PC. Autologous Chondrocyte Implantation in chondral defects of the knee with a type I/III collagen membrane; a prospective study with a 3 year follow-up. Arthroscopy. 2007;23:381-7.
-
22Steinwachs MR, Gille J, Volz M, Anders S, Jakob R, De Girolamo L, et al. Systematic Review and Meta-Analysis of the Clinical Evidence on the Use of Autologous Matrix-Induced Chondrogenesis in the Knee. Cartilage. 2019 Sep 11:1947603519870846.
-
23Armiento AR, Stoddart MJ, Alini M, Eglin D. Biomaterials for articular cartilage tissue engineering: learning from biology. Acta Biomateralia. 2018;65:1-20.
-
24Chenite A, Chaput C, Wang D, Combes C, Buschmann MD, Hoemann CD, et al. Novel injectable neutral solutions of chitosan form biodegradable gels in situ. Biomaterials. 2000;2155-61.
-
25Stanish WD, McCormack R, Forriol F, Mohtadi N, Pelet S, Desnoyers J, et al. Novel scaffold-based BST-CarGel treatment results in superior cartilage repair compared with microfracture in a randomized controlled trial. J Bone Joint Surg Am. 2013;95:1640-50.
-
26Shive MS, Stanish WD, McCormack R, Forriol F, Mohtadi N, Pelet S, et al. BST-CarGel® Treatment Maintains Cartilage Repair Superiority over Microfracture at 5 Years in a Multicenter Randomized Controlled Trial. Cartilage. 2015;6:62-72.
-
27Pipino G, Risitano S, Alviano F, Wu EJ, Bonsi L, Vaccarisi DC, et al. Microfractures and hydrogel scaffolds in the treatment of osteochondral knee defects: a clinical and histological evaluation. J Clin Orthop Trauma. 2019;10:67-75.
Descargar artículo:

Licencia:
Este contenido es de acceso abierto (Open-Access) y se ha distribuido bajo los términos de la licencia Creative Commons CC BY-NC-ND (Reconocimiento-NoComercial-SinObraDerivada 4.0 Internacional) que permite usar, distribuir y reproducir en cualquier medio siempre que se citen a los autores y no se utilice para fines comerciales ni para hacer obras derivadas.
Comparte este contenido
En esta edición
- Update on the treatment of focal cartilage lesions of the knee
- Comparison of the intraarticular injection of platelet rich plasma (PRGF®) and hyaluronic acid (Hyalone®) in the treatment of chondral lesions: a randomized, prospective clinical study
- Microfractures or bone marrow stimulation (BMS): evolution of the technique
- Fresh osteochondral graft. Indications, surgical technique and scientific evidence
- Autologous matrix-induced chondrogenesis (AMIC)
- Instant CEMTRO Cell (ICC), high density autologous chondrocytes implantation
- Regeneration of joint cartilage: perspectives and future
- Autologous chondrocyte implant surgery of the knee
- Full thickness chondral ulcers of the patella and femoral condyle of the knee
Más en PUBMED
Más en Google Scholar
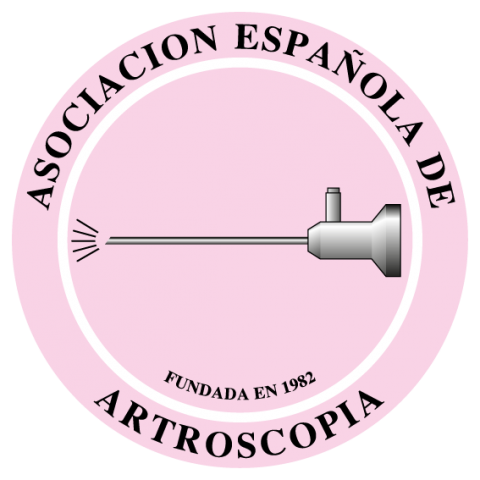

Revista Española de Artroscopia y Cirugía Articular está distribuida bajo una licencia de Creative Commons Reconocimiento-NoComercial-SinObraDerivada 4.0 Internacional.